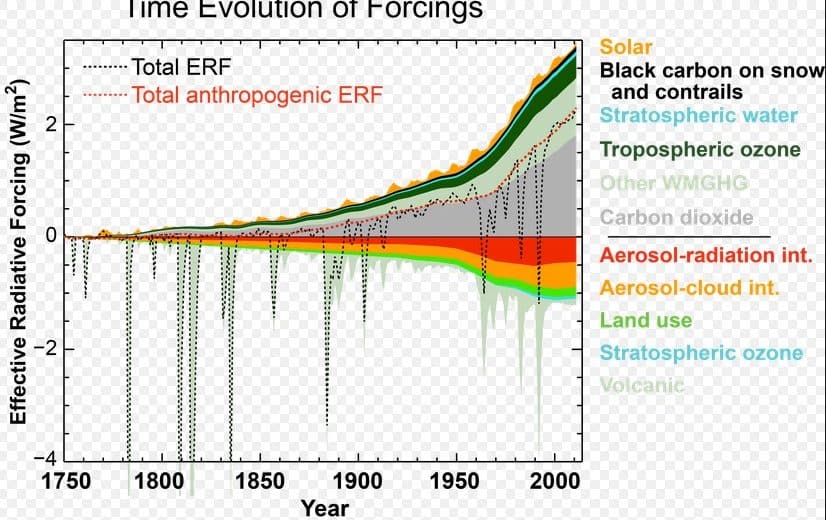
The state of the global climate is one of general stability, engendered by a balance existing between the coupled components of the global climate system. The amount of incoming solar radiation is balanced by the amount of outgoing terrestrial radiation (section 1.2.3), so that the Earth neither continues indefinitely to heat up nor cool down. The Earth’s climate is said to exist in equilibrium. When the climate system responds to radiative forcing (see section 2.3), this equilibrium is temporarily upset and a discrepancy between incoming and outgoing radiation exists. In an attempt to restore equilibrium, the global climate subsequently alters by either heating up or cooling down, depending on the direction of initial forcing.
Although the climate system is in balance, that balance is dynamic, ever-changing. The system is constantly adjusting to forcing perturbations and, as it adjusts, the climate alters. A change in any one part of the climate system will have much wider consequences as the initial effect cascades through the coupled components of the system. As the effect is transferred from one sub-component of the system to another, it will be modified in character or in scale. In some cases it will be amplified (positive feedback), in others, it may be reduced (negative feedback) (Cess & Potter, 1988). It is easiest to understand the concept of feedback by way of an example, the ice-albedo feedback.
Consider an Earth warmed as a result of increased radiative forcing, due, say, to changes in the orbital configuration of the Earth-Sun system. As the Earth’s surface heats up, some of the ice at high latitudes begins to melt, exposing either bare ground or ocean, both of which have lower albedos (reflectivities) than ice. With a lower albedo, the exposed surfaces reflect less incident solar radiation, and the enhanced absorption causes further heating. Further rises in temperature initiate further melting of snow and ice, with further exposure of more energy-absorbent terrain. Thus a cyclic chain reaction of cause and effect is established, with each effect acting as the cause for the next step. This climatic phenomenon is called the ice-albedo feedback (Cubasch & Cess, 1990) and is an example of a positive feedback. The response to primary climatic forcing acts as a secondary forcing mechanism in the same direction as that of the initial forcing factor. Positive feedback augments the climatic response to forcing.
Negative feedback occurs when the response to primary climatic forcing acts as a secondary forcing mechanism in the opposite direction to that of the initial forcing factor. Negative feedback reduces the climate response to forcing. One example of a negative feedback due to increased radiative forcing would be cloud formation (Cubasch & Cess, 1990). As the Earth warms, so the rate of evaporation from the (warmer) oceans increases, supplying the atmosphere with more water vapour conducive to enhanced cloud formation. With greater global cloud coverage, more incident radiation is reflected, reducing radiative forcing and leading to a lowering of the global temperature.
This simple picture of the cloud feedback is, however, complicated by the fact that clouds also serve to trap terrestrial infrared radiation, augmenting the greenhouse effect, and thus act as a positive feedback, also, to increased radiative forcing. Numerical modelling has not been able to determine with any degree of certainty whether positive cloud feedbacks outweigh negative ones, or vice versa. Much depends upon the altitude of the cloud and the cloud type (Charlson et al., 1987; Wigley, 1989). High level clouds are expected to have a net positive feedback, with the effect of long-wave radiation absorption outweighing albedo effects. Clouds at high altitudes exist in colder air and tend to emit less radiation generating a stronger greenhouse effect. Low level clouds, on the other hand, probably have a net negative feedback effect.
There are many other feedback effects which have the potential to influence global climate in response to some initial radiative forcing. These may be found to operate within and between all components of the climate system. Any forcing mechanism which affects the amount of water vapour in the atmosphere will initiate a cloud feedback process. Water vapour, itself, is also a greenhouse gas and forcing perturbations will initiate a water vapour feedback (Cubasch & Cess, 1990; Gates et al., 1992)). Changes in ocean chemistry may occur as a result of primary climatic forcing. For example, warmer water stores less dissolved carbon dioxide, which then remains in the atmosphere to further enhance the greenhouse effect – a positive feedback. Circulation changes within the oceans will also introduce feedback processes affecting the transfer of heat, moisture and momentum (section 2.6.4). Changes in the surface vegetative cover, which has a marked effect on the Earth’s albedo (see section 1.2.3), are likely to have feedback effects on the Earth’s climate (Melillo et al., 1990)
Leave a Reply