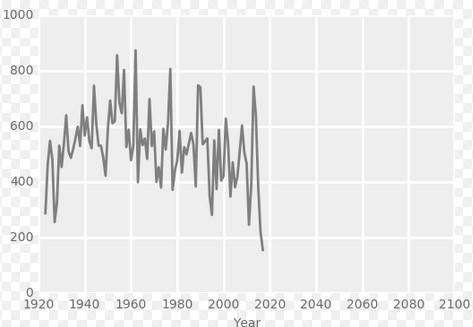
All models must simplify what is a very complex climate system. This is in part due to the limited understanding that exists of the climate system, and partly the result of computational restraints. Simplification may be achieved in terms of spatial dimensionality, space and time resolution, or through parameterisation of the processes that are simulated.
The simplest models are zero order in spatial dimension. The state of the climate system is defined by a single global average. Other models (see section 4.4) include an ever increasing dimensional complexity, from 1-D, 2-D and finally to 3-D models. Whatever the spatial dimension of a model, further simplification takes place in terms of spatial resolution. There will be a limited number of, for example, latitude bands in a 1-D model, and a limited number of gridpoints in a 2-D model. The time resolution of climate models varies substantially, from minutes to years depending on the nature of the models and the problem under investigation.
In order to preserve computational stability, spatial and temporal resolution have to be linked. This can pose serious problems when systems with different equilibrium time scales have to interact as a very different resolution in space and time may be needed.
Parameterisation involves the inclusion of a process as a simplified (sometimes semi-empirical) function rather than an explicit calculation from first principles. Subgridscale phenomena such as thunderstorms, for example, have to be parameterised as it is not possible to deal with these explicitly. Other processes may be parameterised to reduce the amount of computation required.
Certain processes may be omitted from the model if their contribution is negligible on the time scale of interest. For example, there is no need to consider the role of deep ocean circulation whilst modelling changes over time scales of years to decades. Some models may handle radiative transfers in great detail but neglect or parameterise horizontal energy transport. Other models may provide a 3-D representation but contain much less detailed radiative transfer information.
Given their stage of development, and the limitations imposed by incomplete understanding of the climate system and computational constraints, climate models cannot yet be considered as predictive tools of future climate change. They can, however, offer a valuable window on the workings of the climate system, and of the processes that have influenced both past and present climate.
Leave a Reply